Understanding Wavelength: Measurement Techniques
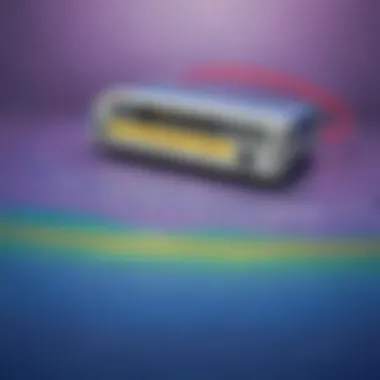
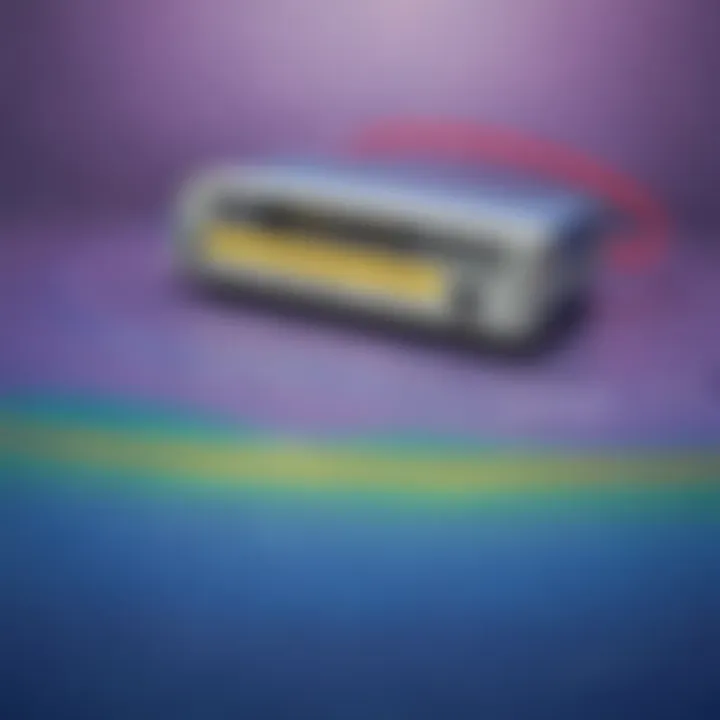
Intro
Wavelength is a fundamental concept in various scientific fields. Its measurement is critical in understanding waves, including light and sound. This article explores the methodologies for measuring wavelength. Both traditional and modern techniques will be discussed. Wavelength impacts fields like physics, chemistry, and communication technologies. Understanding this topic can be beneficial for educators, parents, and curious learners.
Measuring wavelength involves both theoretical knowledge and practical techniques. As we go deeper into the subject, it is important to consider how these measurements affect everyday technology and scientific research.
Measurement Techniques
Wavelength measurement encompasses a variety of techniques. These methods range from classic approaches to advanced technologies. Each technique has its own set of applications and significance.
Traditional Methods
- Spectroscopy: A common method used to determine wavelength. Spectroscopy analyzes the interaction between light and matter. It provides valuable data in different disciplines.
- Ruler Method: This basic method involves measuring the distance between wave peaks. Though simple, it requires precision to ensure accurate results.
Modern Techniques
- Interferometry: This technique uses the principle of interference. By analyzing patterns formed when waves overlap, precise wavelength measurements can be made.
- Laser Measurement: Lasers provide a stable and coherent source of light. Modern instruments using lasers can measure wavelengths with high accuracy.
The choice of method often depends on the application and the required precision. Understanding these techniques enhances one's ability to engage with the scientific process.
Importance of Wavelength Measurement
The measurement of wavelength is not merely a technical necessity; it is foundational across various scientific domains.
In physics, it aids in understanding electromagnetic spectra and quantum mechanics. Chemistry relies on wavelength measurement for spectroscopy, vital for analyzing molecular structures.
Applications in Communication
- Wavelength is crucial in telecommunication systems. Different wavelength bands correspond to specific frequencies used in data transmission.
- Knowledge of wavelength directly affects the design and efficiency of optical fiber networks.
Educational Value
- Teaching about wavelength measurement fosters critical thinking. Hands-on experiments can enhance students' comprehension and retention.
- Engaging students in practical applications encourages a deeper interest in science.
"Wavelength measurement is essential in bridging academic concepts with real-world applications."
Through these efforts, students and learners can connect theory with practice, leading to a more profound understanding of scientific principles.
Closure
Preface to Wavelength
Wavelength is a fundamental concept in the study of waves, especially in physics and optics. Understanding this term allows researchers and students to grasp a variety of scientific phenomena. Wavelength refers to the distance between consecutive peaks of a wave. This concept is important because it directly relates to the behavior of different types of waves, including sound waves, electromagnetic waves, and light waves.
The measurement of wavelength helps scientists quantify how energy is transported through different mediums. It serves as a basis for many applications, from telecommunications to environmental science.
Definition of Wavelength
In simplest terms, wavelength is the spatial period of a periodic wave. It is usually denoted by the Greek letter lambda (ฮป). Wavelength is measured in meters, and it indicates the distance between identical points on consecutive waves. For example, if one were to measure from one peak of a wave to the next peak, that measurement would represent the wavelength.
Mathematically, wavelength can be defined in relation to frequency and the speed of the wave. The equation used is:
[ \lambda = \fracvf ]
Where:
- ( \lambda ) is the wavelength,
- ( v ) is the speed of the wave,
- ( f ) is the frequency of the wave.
This relationship shows how wavelength, frequency, and wave speed are interconnected, making it essential for various scientific calculations.
Significance of Wavelength in Science
Wavelength is crucial across many scientific disciplines. In physics, it plays a key role in understanding the behavior of light. Different wavelengths correspond to different colors of light. This phenomenon is observed in technologies such as prisms and spectrometers, which separate light into its constituent colors based on wavelength.
In chemistry, wavelength measurements can provide information about molecular structures and reactions. Instruments like spectrophotometers utilize specific wavelengths to analyze substances, helping chemists understand chemical properties and interactions.
Moreover, in biology, the study of wavelengths is vital for techniques such as fluorescence microscopy. Here, specific wavelengths excite fluorescent dyes, allowing for the visualization of cells and biological processes.
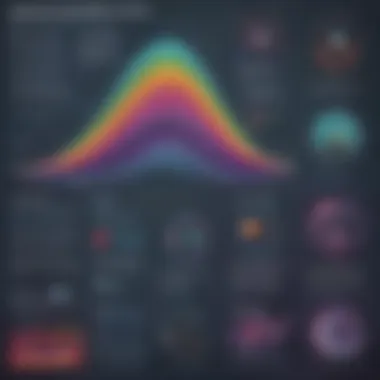
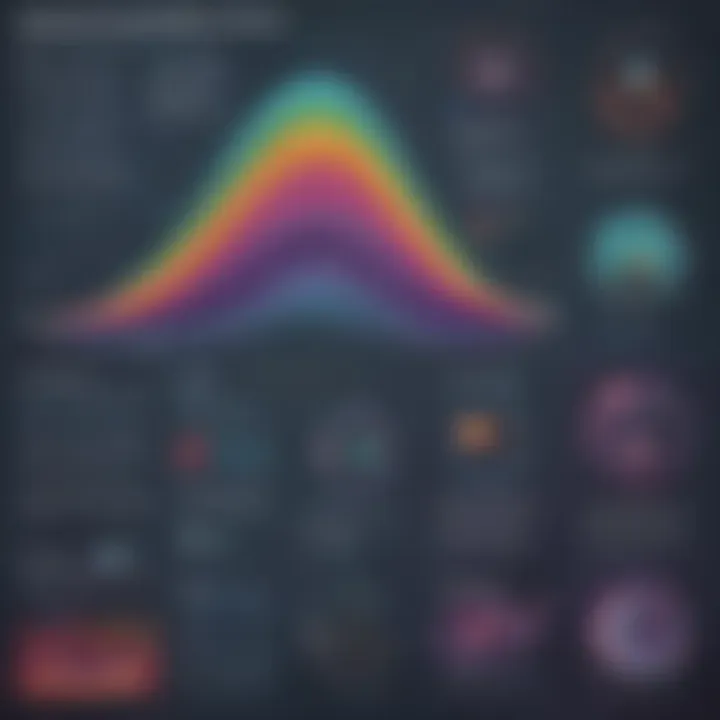
In summary, wavelength is more than just a measure of distance; it is a pivotal element that informs research, innovation, and education across diverse scientific fields. Understanding its definition and significance promotes a deeper appreciation and knowledge of the natural world.
"Wavelength is not just a distance; it is a gateway to understanding the universe's complexities.''
Exploring wavelength opens pathways to new technologies and scientific advancements. As we delve deeper into measurement techniques, this fundamental concept will serve as the backbone of our exploration.
Fundamental Concepts
The concept of wavelength is a cornerstone in the study of waves, particularly in the fields of physics and engineering. Understanding these fundamental concepts is essential for anyone interested in how waves interact with their environments. This section aims to elucidate the critical aspects of light waves and the relationships that bind wavelength, frequency, and speed.
Understanding Light Waves
Light waves are electromagnetic waves that are visible to the human eye. They carry energy and travel through a vacuum or any medium. The speed of light in a vacuum is approximately 299,792 kilometers per second. This speed can change when light travels through materials such as glass or water, which is significant because it affects how we perceive and utilize light in various applications.
The behavior of light can be described using various properties:
- Wavelength: This is the distance between consecutive peaks or troughs in a wave. The wavelength determines the color of visible light. For instance, violet light has a shorter wavelength while red light has a longer wavelength.
- Frequency: This refers to the number of wave cycles that pass a given point per second, measured in hertz (Hz). Higher frequencies correspond to shorter wavelengths.
- Amplitude: This describes the height of the wave, influencing the brightness of the light. Higher amplitude means brighter light.
All these properties interact to create the range of phenomena we observe in the natural world, from the colors in a rainbow to the functioning of lasers.
Relationships Between Wavelength, Frequency, and Speed
The relationship between wavelength, frequency, and speed is encapsulated in the formula:
[ c = \lambda \cdot f ]\
Where:
- c is the speed of light
- \lambda (lambda) is the wavelength
- f is the frequency
This formula shows that as the wavelength increases, the frequency decreases, and vice versa. It underscores a fundamental principle in wave physics: wavelength and frequency are inversely related. This understanding is crucial, especially in areas like telecommunications, where different wavelengths are used for various services like radio, television, and mobile communications.
Moreover, the speed of light remains constant in a vacuum, but can slow down in different media. This phenomenon affects how light bends or refracts, which is pivotal in lens design and various optical devices.
In summary, the fundamental concepts related to wavelength provide a solid foundation for exploring more complex topics in wave physics. This understanding can lead to practical applications in technology, education, and environmental science.
Traditional Methods of Wavelength Measurement
Traditional methods for measuring wavelength hold significant importance in various scientific contexts. These techniques provide foundational knowledge essential for understanding more complex systems of measurement. They often emphasize mathematical reasoning and empirical observations, which form the bedrock of wavelength studies.
Mathematical approaches invite a rigorous examination of the relationships within wave phenomena. They serve as educational tools, demonstrating principles like the relationship between wavelength and frequency, making this a crucial area of study for students and researchers alike.
Additionally, traditional measurement techniques, such as the usage of prisms and diffraction gratings, have historical significance. They not only illustrate core scientific concepts but also pave the way for the development of contemporary technologies. Understanding these traditional methods prepares educators, parents, and students for further inquiry into advanced measurement methodologies.
Using Mathematical Calculations
Mathematical calculations play a vital role in wavelength measurement. The fundamental equation of light, represented by the formula [\lambda = \fraccf], indicates that wavelength ([\lambda]) is the speed of light ([c]) divided by frequency ([f]). This simple formula leads to significant insights into wave behavior and properties. It enables scientists to relate the wavelength to its associated frequency, facilitating direct measurement of electromagnetic waves.
By applying simple algebraic manipulation, one can predict various properties of waves, including energy and momentum. As students engage with these calculations, they cultivate critical thinking and problem-solving skills that are beneficial in various areas of study. These calculations offer clarity and a way to quantify theories, making them indispensable in both classroom and research settings.
Prism and Diffraction Grating Techniques
Prisms and diffraction gratings are essential tools in wavelength measurement, showcasing the principles of light behavior. Prisms work by refracting light and separating it into its constituent colors. This separation produces a spectrum that can be observed and analyzed. The angle of refraction directly correlates with wavelength, allowing scientists to infer values of wavelength based on standard measurements.
Diffraction gratings work on a similar principle, utilizing multiple slits to produce interference patterns. These patterns occur due to the wave nature of light, revealing specific constructive and destructive interference properties. The angles at which bright spots appear help researchers calculate the wavelength of the incident light. Using these techniques provides precise measurements and has implications in fields such as telecommunications and spectroscopy.
"Understanding these traditional techniques enriches a student's grasp of fundamental scientific principles and aids in the development of modern technologies."
In sum, both mathematical techniques and physical tools like prisms and diffraction gratings lay the groundwork for deeper exploration into more advanced measurement techniques. They offer not only measurements but also a comprehensive understanding of wavelength's role across scientific disciplines.
Modern Techniques for Measuring Wavelength
Measuring wavelength accurately is essential in various scientific fields, including physics, chemistry, and environmental science. Modern techniques for measuring wavelength have significantly improved the precision and efficiency of these measurements. As technology advances, new methods emerge that not only enhance the accuracy of wavelength measurements but also broaden the range of applications available to scientists and researchers. This section examines two prominent modern techniques: spectroscopy and laser interferometry.
Spectroscopy

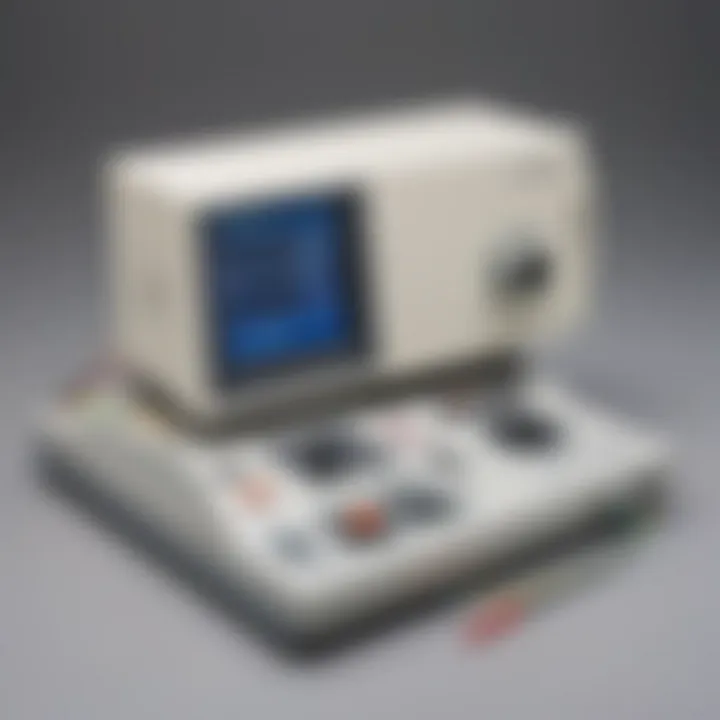
Spectroscopy is a powerful technique used to analyze the interaction of light with matter. By examining the light emitted or absorbed by substances, scientists can determine the wavelengths of that light. Spectroscopy is vital in fields such as chemistry for identifying substances, in astronomy for analyzing the composition of stars, and in environmental science for monitoring pollutants.
The core principle of spectroscopy lies in the fact that each element absorbs and emits light at specific wavelengths. This phenomenon leads to the creation of spectra, which are unique to each element. A spectroscope or spectrometer can be used to disperse light into its constituent wavelengths, enabling detailed analysis. Techniques such as ultraviolet-visible (UV-Vis) spectroscopy, infrared (IR) spectroscopy, and Raman spectroscopy each focus on different segments of the light spectrum, providing a versatile toolkit for measurement.
"Spectroscopy allows the extrapolation of information from light interactions, revealing material properties not visible by other means."
Using spectroscopy, researchers can conduct several key analyses:
- Quantitative Analysis: Determining the concentration of substances in a sample by measuring the intensity of light absorption.
- Qualitative Analysis: Identifying substances based on their unique spectral fingerprints.
- Kinetics Studies: Observing reactions in real time, allowing insights into the speed and mechanisms of chemical processes.
Laser Interferometry
Laser interferometry is another modern technique employed to measure wavelengths with high precision. This method relies on the principles of interference patterns created by overlapping laser beams. By carefully controlling the conditions, researchers can measure minute changes in distance and wavelength.
Interferometry is particularly useful in fields where high accuracy is crucial, such as astrophysics, material science, and quantum optics. Adjustments in wavelength can reveal changes in distance to an accuracy of fractions of the wavelength itself, enabling measurements on a nanometer scale.
The principle behind laser interferometry is relatively straightforward. When two laser beams are combined, they create an interference pattern, which consists of alternating light and dark bands. By analyzing these patterns, scientists can deduce information about wavelength and phase shifts.
Some important applications of laser interferometry include:
- Metrology: Calibration of measuring devices due to its high-precision measurements.
- Seismology: Monitoring ground movement, providing insights into seismic activity.
- Optical Coatings: Inspecting the quality of optical components, ensuring they meet stringent specifications.
In summary, modern techniques like spectroscopy and laser interferometry have transformed wavelength measurement. These methods offer enhanced accuracy and adaptability, critical for advancing knowledge in multiple scientific domains.
Wavelength Measurement Tools
Wavelength measurement tools are essential in both scientific research and practical applications. Understanding how these tools function can provide insight into many fields, including physics, chemistry, and educational contexts. Their significance lies in their ability to accurately quantify light properties, which directly influences the analysis of various phenomena and technologies. Having the right instrument can enhance the accuracy of measurements and broaden the scope of scientific inquiry.
Spectrometers
Spectrometers are devices that measure the intensity of light at different wavelengths. They are critical in various scientific disciplines. For instance, in chemistry, spectrometers help determine the composition of substances, allowing researchers to analyze spectral lines that indicate the presence of specific chemicals. Their operation typically involves dispersing light into its component wavelengths, which can be achieved through diffraction gratings or prisms.
The data collected can be visualized in a spectrum, showing how much light is available at each wavelength. This information is essential for tasks like identifying materials, measuring concentrations, and studying electronic transitions in atoms.
In an educational setting, using spectrometers can engage students by providing hands-on experiences. They can experiment with different light sources and analyze how the spectrum changes, reinforcing fundamental concepts of light and wavelength.
Photodetectors
Photodetectors play a crucial role in converting light into an electrical signal. These devices are sensitive to light intensity and can determine the wavelength of incoming light. They are used in a variety of applications, from telecommunications to environmental monitoring.
Common types include photodiodes and photomultiplier tubes. Photodiodes offer quick response times and are often used in digital devices, while photomultiplier tubes are used in applications that require detection of very low levels of light.
In educational activities, photodetectors can help students understand the interactions between light and matter. By measuring light intensity and wavelength, learners gain practical knowledge about how these devices function and their significance in real-world applications.
Oscilloscope Applications
Oscilloscopes are instruments that graph electrical signals over time. While they are primarily used for voltage measurements, they can also be used to analyze light waves by pairing them with photodetectors. This combination allows one to visualize changes in light intensity as a function of time, which can be particularly useful in various research scenarios.
In descriptive terms, oscilloscopes provide a time-based view of wavelength data, enabling scientists to study periodic phenomena. This makes them valuable in fields such as engineering and physics.
Teaching through oscilloscopes can break down complex concepts into more digestible portions, allowing students to visualize waveforms directly.
"Understanding how to use oscilloscopes can significantly enhance a student's grasp of waveform behavior, thus facilitating deeper insight into wavelength measurement."
The Role of Wavelength in Education
Wavelength measurement is not just for scientists and engineers; it has a significant role in education that can enhance the learning experience of students. Understanding concepts related to wavelength is crucial for grasping key principles in physics, chemistry, and telecommunications. These scientific foundations have become increasingly relevant in today's technology-driven world. More than mere academic interest, the study of wavelength contributes to critical thinking and problem-solving skills among students.
Educators can create an engaging learning environment by integrating these concepts into the curriculum. This not only builds knowledge but also fosters a sense of intrigue about the natural world.
Incorporating Wavelength Concepts in Curriculum
Integrating wavelength concepts into educational curriculum helps to contextualize theoretical knowledge. Various subjects can incorporate the topic of wavelength uniquely. For instance:
- Physics: Discuss the electromagnetic spectrum, detailing how different wavelengths affect energy and frequency.
- Chemistry: Analyze how wavelength is involved in atomic and molecular spectra, leading to a deeper understanding of chemical reactions and properties.
- Environmental Sciences: Study the impact of light waves in ecosystems, such as photosynthesis in plants.
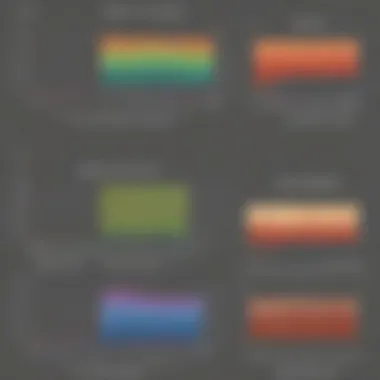
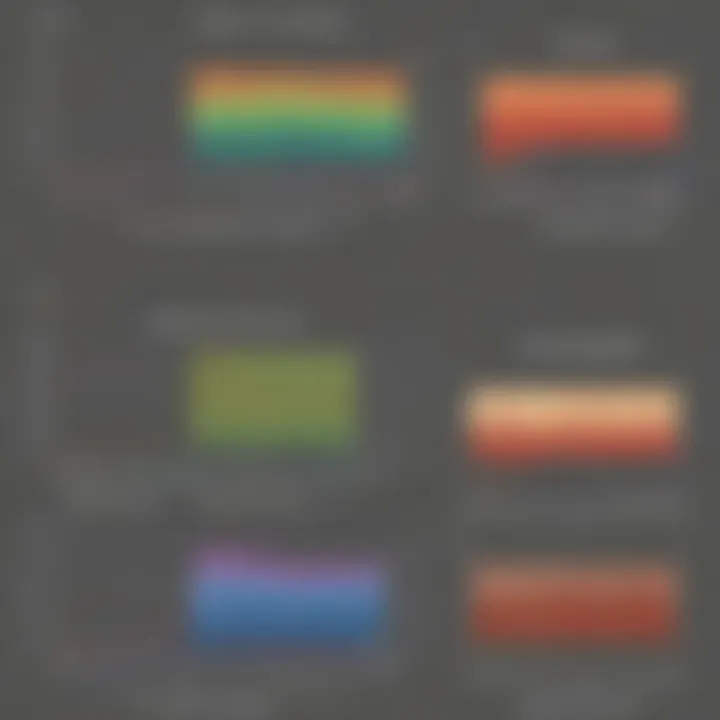
When students learn about how wavelengths apply directly to their lives, they relate better to the material. Assignments can include practical exercises like measuring wavelengths using simple home experiments.
Engaging Activities for Kids
Engaging kids in hands-on activities related to wavelength can spark curiosity. Here are several ideas:
- Prism Experiment: Use a glass prism to explore how light refracts into different colors. By measuring the angles, students can relate to the concepts of wavelengths in a visual manner.
- Build a Spectroscope: Use cardboard tubes and diffraction gratings to create a simple spectroscope. Kids can observe and measure different spectra from various light sources.
- Wavelength Relay: Organize a relay game where students must identify colors of light according to wavelengths. This combines physical activity with educational content in a fun manner.
"Through practical applications and engaging activities, students can develop a robust understanding of wavelength that extends beyond the classroom."
Applications of Wavelength Measurement
Understanding how to measure wavelength is critical in many fields today. This measurement has practical applications that influence our daily lives, technology, and environmental understanding. Several sectors benefit from precise wavelength measurements, enabling advancements in communication and environmental science.
Telecommunications and Signal Processing
Wavelength plays a crucial role in telecommunications. The efficiency of data transmission heavily relies on wavelength. In fiber optic cables, light travels in different wavelengths, carrying information in the form of binary code. By utilizing varying wavelengths, multiple data streams can function simultaneously without interference. This technology, known as wavelength-division multiplexing, enhances bandwidth and speeds up communication systems dramatically.
In signal processing, wavelength influences how signals are modulated and decoded. Techniques in wavelength measurement assist in ensuring that signals are transmitted accurately, reducing the chances of error. Accurate wavelengths lead to clearer audio and visual content, highly desired in media and entertainment industries.
"Without precise wavelength measurement, modern telecommunications as we know it would not be possible."
Environmental Monitoring
Wavelength measurement is essential for monitoring environmental conditions. It helps in assessing air and water quality. For example, sensors can detect pollutants by measuring the wavelengths of light absorbed by different chemicals. This application is critical for ensuring safe environments and public health.
Remote sensing technology relies on wavelengths to gather data about Earth's surface. Satellites use spectral information to determine vegetation health, soil conditions, and changes in land use. By analyzing wavelengths reflected back from the surface, scientists can derive vital information for climate studies and resource management.
Challenges in Wavelength Measurement
Understanding the challenges in wavelength measurement is crucial to grasp the nuances involved in this scientific endeavor. These challenges can affect the accuracy and reliability of measurements, which in turn influences research outcomes across various fields. As we continue to develop new technologies and methodologies for wavelength measurement, it is essential to recognize these limitations and seek improvements.
Limitations of Current Techniques
One primary limitation of current techniques for measuring wavelength lies in their precision and accuracy. Traditional methods, such as using prisms or diffraction gratings, can sometimes lead to measurement errors due to environmental factors. Changes in temperature, humidity, or even air pressure can distort the results, leading to unreliable data.
Another significant limitation is the resolution of the equipment used. Many spectrometers cannot distinguish between wavelengths that are very close together. This limitation can become more pronounced when studying complex materials that emit spectra with overlapping wavelengths. Additionally, there is often a trade-off between sensitivity and speed. Instruments that are highly sensitive may take longer to provide accurate readings, which can be a disadvantage in real-time applications.
Moreover, the calibration of equipment is a recurring challenge. Calibration drift over time can introduce errors in measurements. Devices need regular maintenance and recalibration to ensure they operate within acceptable ranges. This requirement adds to the operational costs and logistical challenges involved in wavelength measurement over time.
Improvements and Innovations Needed
To address these limitations, advancements in technology and innovative techniques are essential. One potential area of improvement is the development of more precise and sensitive measuring instruments. For example, integrating advanced materials with superior optical properties can enhance the resolution of spectrometers, enabling them to distinguish closely spaced wavelengths effectively.
Improvements in data processing algorithms could also help in refining measurements. Adaptive algorithms that consider environmental variables in real-time can compensate for distortions caused by external factors. This approach may lead to more accurate and reliable measurements.
The introduction of photonic technologies, such as optical frequency combs, represents a frontier in wavelength measurement. These systems provide a consistent frequency reference, which could significantly enhance measurement accuracy.
Moreover, increasing accessibility to higher-end measurement tools will be beneficial for educational and research institutions. This can facilitate hands-on learning and practical experience in wavelength measurement techniques, making these concepts more tangible for students and educators alike.
"As we untangle the complexities of wavelength, our tools must evolve to keep pace with scientific discovery."
Understanding and overcoming these challenges in wavelength measurement will not only advance scientific research but enhance educational practices as well. By engaging with the latest innovations, educators can ensure that the next generation is equipped to tackle the evolving landscape of science.
Culmination
The conclusion serves a pivotal role in summarizing the relevance and importance of the measurement of wavelength in various scientific fields. It is an opportunity to reflect on how these measurements influence both theoretical understanding and practical applications. In a time where technology evolves rapidly, grasping the concept of wavelength and its measurement becomes necessary for advancements in diverse fields such as telecommunications, environmental monitoring, and educational frameworks.
Summary of Key Points
In this article, we explored several critical topics regarding wavelength measurement:
- Definition of Wavelength: Understanding what wavelength is and its implications in physics and other sciences.
- Measurement Techniques: The detailed comparison between traditional methods like mathematical calculations and modern approaches such as spectroscopy.
- Tools Used: A look into the various instruments, including spectrometers and photodetectors, essential for accurate wavelength measurements.
- Applications: How wavelength measurement aids in practical fields, including telecommunications and environmental monitoring.
- Challenges: Insight into the limitations faced and the innovations necessary to overcome them.
This synthesis serves not only to reaffirm the importance of wavelength measurement but also encourages further inquiry into how these methods can evolve and improve.
Future Directions in Wavelength Measurement
As we look toward future developments in wavelength measurement, certain trends appear prominent:
- Increased Precision: Advances in technology may enable even more precise measurements, essential for applications requiring high accuracy, such as quantum computing.
- Integration of AI: Artificial intelligence might enhance data analysis from wavelength measurements in real-time, improving industries like telecommunication through better signal processing.
- Environmental Applications: With climate change being a pressing global issue, more focus is likely to be placed on using wavelength measurements to monitor pollutants and understand ecological changes.